At the nanoscale, the smallest structural differences can have a big impact.
When materials and devices are measured in nanometers, the placement and orientation of their individual atoms determine the way they work. For example, standard DNA’s double helix shape always twists to the right, thanks to the way the sugars in its molecular backbone stack on top of one another. While “left-handed” DNA is also possible, such a helix would be incompatible with the rest of the body’s protein-making molecular machinery.
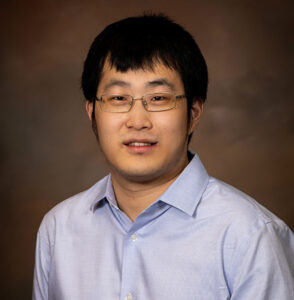
This property of twisted structures, known as chirality, is found throughout nature, but is difficult to design and fabricate at wafer scale: the size necessary to be compatible with the mass-manufacturing processes used in the computer chip industry. Advanced nanomanufacturing techniques can produce chiral structures in small quantities, but achieving the precision and consistency required to make them useful on the macroscale remains a challenge.
Weilu Gao, assistant professor in the Department of Electrical and Computer Engineering at the University of Utah’s John and Marcia Price College of Engineering, has led a new study that shows a pair of techniques that could solve this problem.
Published in the journal Nature Communications, Gao and his colleagues from Rice University and Japan have demonstrated two methods for achieving engineered chirality in carbon nanotube films, known as “twist stacking” and “mechanical rotation,” which are extensions of the deposition technique Gao previously developed known as “controlled vacuum filtration” or CVT.
In the researchers’ new study, carbon nanotubes are deposited into thin films with chiral architectures in controlled manners. The obtained thin films have different responses to circularly polarized light of opposite handednesses, which is called circular dichroism. Those films could be key to new photonic and optoelectronic devices, such as chiral optical emitters, chiral optical sensors, and chiral photodetectors. Those films also provide opportunities for exploration of new phenomena for broader applications, including non-optical effects, such as spintronics.
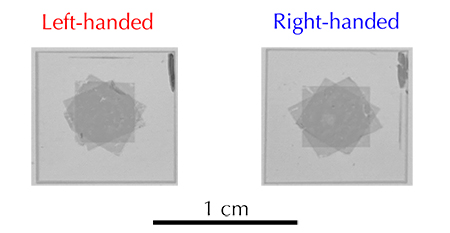
Consisting of a single layer of carbon atoms rolled up into a tube-like shape, carbon nanotubes can be thought of as one-dimensional nanowires with highly desirable physical, optical, and electromagnetic properties, and are promising for many electronic, photonic, and optoelectronic applications. However, in the macroscopic world, practical applications might require trillions of individual carbon nanotubes to be aligned in the same direction at wafer scale, an organizational challenge made more difficult by their minuscule size — a standard carbon nanotube is roughly 5 million times thinner than a pencil. With CVT, carbon nanotubes are suspended in water, then flowed through a filter membrane. Grooves on the membrane form a template for aligning carbon nanotubes into the desired orientation. Once dried, the filter can be chemically dissolved, leaving a thin film of highly ordered carbon nanotubes at the wafer scale.
In their new study, Gao and his co-authors put a literal twist on top of this CVT method in two different ways. “When I prepared my research statement when looking for academic jobs, I made such a prediction that twisted stacks of aligned carbon nanotubes prepared using the CVT method were able to show chiro-optical response” Gao says, “Now, we have proven such prediction through experiments. This is a joyful moment for a scientist.”
With “twist stacking,” the researchers produced multiple carbon nanotube films with traditional CVT, then layered them such that the overall orientation of the carbon nanotubes in each were rotated relative to one another. With “mechanical rotation,” the researchers spun the CVT filter as carbon nanotubes accumulated, producing a single film with a tornado-like pattern throughout the structure.
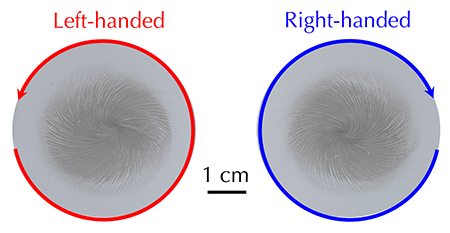
The researchers also demonstrated the ability to engineer the films’ chiro-optical properties by fine-tuning both the twist stacking and mechanical rotation fabrication techniques. For the former, the researchers produced stacks of films with different overall properties by changing the degree and direction of rotation between its layers and achieved strong circular dichroism in the deep ultraviolet wavelength range; for the latter, they altered the speed and direction of the filter’s rotation as carbon nanotubes accumulated on it.
In addition, the researchers have developed a theoretical framework to reproduce all experimental observations. “With this framework, we predicted the chiro-optical response of films can be further optimized” says Minhan Lou, a former postdoctoral researcher in Gao’s lab who contributed to the study.
Other members of the research team include Jacques Doumani, Oliver Dewey, Nina Hong, Jichao Fan, Andrey Baydin, Keshav Zahn, Yohei Yomogida, Kazuhiro Yanagi, Matteo Pasquali, and Riichiro Saito. Their study has been selected as a featured article in collections of “Inorganic and physical chemistry” and “Materials science and chemistry” of Nature Communications.
The research was supported by the National Science Foundation through Grant Nos. 2321366 and 2235276; the PIRE U.S.-Japan Program through Grants No. 2230727 (US National Science Foundation) and JPJSJRP20221202 (Japan); the Robert A. Welch Foundation through Grants Nos. C-1509 and C-1668; the Air Force Office of Scientific Research through Grant No. FA9550-22-1-0382; the Chan Zuckerberg Initiative through Grant No. WU-21-357; the Department of Energy through Grant No. DE-AR0001015; JSPS KAKENHI through Grants Nos. JP20H02573, JP21H05017, JP22H05469, JP23H00259, and JP22H00283; JST CREST through Grant No. JPMJCR17I5; and Taiwan’s Yushan Fellow Program.
First published by the John and Marcia Price College of Engineering.
Learn more about Electrical and Computer Engineering faculty research topics and discover ways to get involved in undergraduate research.